Hydrogen will need to be transported over varying distances, from points of production to intermediaries and end-users. Today, there is a high co-location of production and demand for GH2 in hydrogen clusters/valleys, which means that GH2 is mostly produced using on-site electrolysers in areas with favourable conditions for producing renewable energy.1 In the future, however, economies of scale and improved safety standards may make it profitable and safe to produce hydrogen in large installations and then distribute it more broadly to different users.2 In addition, by 2050, 3% of the global final energy demand is expected to be traded as hydrogen.3 As both production volumes and transport distances expand to meet increasing global demand, significantly more hydrogen infrastructure will be required to connect GH2 production with demand centres and international markets.
The challenge
Centralized production of GH2 for domestic use or export requires a hydrogen storage and transportation network infrastructure, including pipelines, ports and terminals, conversion plants and large-scale storage facilities. This infrastructure will be crucial for realizing the potential that GH2 holds for decarbonization (global North) and development (global South). An estimated 55% of cross-border hydrogen transport will be via pipelines4, as they can deliver a continuous flow of gaseous hydrogen (or its derivatives) at relatively high efficiency, while shipping will be used for more flexible distribution over extended distances.5 The necessary infrastructure is, however, almost non-existent at present.
Technical challenges
Transportation and large-scale conversion/reconversion of hydrogen is currently a complex endeavour, threatening the large-scale adoption and competitiveness of GH2. For example, while hydrogen has two-and-a-half times the energy density of natural gas, it is also three times lighter under equivalent conditions, necessitating higher pressures or volumes in supply. This makes it challenging to repurpose existing infrastructure. Moreover, hydrogen’s considerably lower boiling point (at -253°C compared to -162°C for natural gas) complicates transportation. For shipping, hydrogen must first be converted into denser forms using energy-intense processes, such as liquefaction, or it must be transformed into substances like ammonia or synthetic hydrocarbon fuels.6
To construct the relevant transport infrastructure, an estimated spending of USD 200 billion is required by 2030.7 As only about 10% of currently proposed hydrogen investments focus on infrastructure, an investment gap of about 85% exists.
Because transportation costs constitute a significant portion of the overall hydrogen costs8, planning, international cooperation and investment mobilization are urgently needed.
Government planning
Due to the significant lead-times and costs of infrastructure construction, governments need to plan their hydrogen transport networks carefully and integrate them in the wider industrial and energy strategies. Identifying transport routes, solutions and corridors in GH2 road maps will help attract funding by allowing investors to evaluate future market prospects.9
Governments, businesses and investors may also consider no-regret options in hydrogen transport infrastructure. Termed “no-regret” due to their likelihood of avoiding resource wastage, these options are generally characterized by adaptability and low risk. These choices remain beneficial and relevant despite uncertain developments in the hydrogen industry, ensuring valuable investments regardless of market shifts.10
A no-regret vision also needs to reduce the risk of oversizing the future hydrogen network will most likely be smaller than the current natural gas network – by focusing on indispensable demand. Policymakers can identify robust no-regret corridors for early hydrogen transport based on industrial demand, with industrial clusters presenting some of the clearest opportunities.11 Demand in other sectors, such as heating and road transport, is much less certain due to efficient electrification possibilities. Thus, anchoring infrastructure on industrial hydrogen demand is a risk-minimizing strategy that mitigates the risks of oversizing, asset stranding or project abandonment.12
Pipeline (re)construction
Once “no-regret” areas for hydrogen transport are identified, the conversion of the gas grid to hydrogen can be initiated.13 Repurposing part of the existing fossil fuel pipeline network to transport hydrogen can provide new infrastructure while reducing the stranding of obsolete installations in a relatively quick and cost-effective way.14 It offers an advantageous financing case since required CAPEX investments are 65–94% lower than for new, purpose-built hydrogen pipelines15, although the expected lifetime of a repurposed pipeline will likely be shorter than a new one. Another consideration, given the technical obstacles presented by a 100% hydrogen grid, is that synthetic methane can often be transported in unmodified pipelines.16
Nevertheless, constructing dedicated infrastructure will be inevitable to link emerging demand and supply areas. Installing new purpose-built hydrogen pipelines parallel to old gas pipelines can result in costs savings and shorter timelines by leveraging the established right-of-way, siting permits and existing laid groundwork.17
Hydrogen carriers for shipping
Seaborne transportation will enable flexible long-distance hydrogen trade, using three main carriers (see figure below): liquefied hydrogen (LH2), ammonia (NH3), and Liquid Organic Hydrogen Carriers (LOHC). As the market grows, all carriers are expected to see significant cost reductions, particularly LOHC.18 Selecting the best carrier depends on operational factors and costs: LH2 is suitable for high-purity hydrogen when on-site reconversion isn’t feasible, ammonia is cost-effective for small-scale multimodal transport when some infrastructure already exists, while LOHC offers safety and cost-reduction potential. The choice should be tailored to specific supply routes, considering individual circumstances.19
Energy available along the conversion and transport chain in hydrogen equivalent terms, 2030
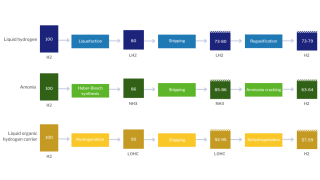
The ultimate dominance of a specific technology depends on factors such as market uptake speed, potential for cost reduction, and the ability to provide a safe and user-friendly solution. This entails significant uncertainty for the future path of long-distance GH2 distribution and trade because infrastructure such as terminals and converters are different for each associated carrier, incurring a risk of technological lock-in and stranded assets.
Conclusion
International coordination will be imperative, as it was for propelling the global liquid natural gas (LNG) market, to provide a clear path forward and prevent delays in infrastructure roll-out, which would hamper GH2 adoption and climate mitigation. Smart planning, clear regulation and meaningful support are therefore all required to mobilize investment in GH2 infrastructure for low-carbon growth in the global South, and decarbonization in the North.
This opinion piece is a snapshot of the GH2 policy toolkit for developing countries being developed by UNIDO, IRENA and IDOS and to be launched at COP28.
Disclaimer: The views expressed in this article are those of the authors based on their experience and on prior research and do not necessarily reflect the views of UNIDO (read more).
Have your say
What is your opinion on the IAP?