Ceramics are intrinsically associated with human development, appearing in the archaeological record as early as 28,000 B.C.E. Ceramics have been used as building materials, containers, decorative objects and in a wide range of infrastructural, technical and cultural artefacts. The ceramics industry comprises various products, including bricks and roof tiles, wall and floor tiles, household ceramics, vitrified clay pipes, sanitary ware, expanded clay for construction purposes, and refractory and abrasive ceramics for industrial applications. Ceramics are also an important component of the world economy. In Europe alone, this industry employs 338,000 people, engages 2,000 companies producing 1,304 million m2 of tiles per year, and contributed to an annual business turnover of 30 billion euros in 2018.1
Despite the relevant role of ceramics in our society, their manufacturing processes come with negative social and environmental impacts. For instance, the production of wall and floor tiles, bricks and roof tiles, and refractories results in 19 Mt of CO2 emissions in the EU annually, while brick accounts for 20 per cent of the world’s black carbon (particulate) emissions, making it one of the most polluting materials on earth. In Asia alone, brick production is estimated to consume more than 110 million metric tonnes of coal per year. Globally, the annual energy consumption for firing ceramics through natural gas is estimated at 182 TWh. Further impacts can be seen in the reassignment of fertile soil; in India, for example, about 272 million metric tonnes of fertile soil are consumed per day for brick manufacturing purposes. Finally, the health-related repercussions of the ceramics industry are most notable in their impact on labourers and surrounding communities. In Dhaka, Bangladesh, for example, bricks production has led to around 2,200 to 4,000 premature deaths and 0.2 to 0.5 million asthma attacks per year. On top of that, many of these labourers come from vulnerable populations and work under exploitative conditions.2
However, ceramics also have the potential to provide environmental benefits. For instance, they could be used to purify industrial wastewater, for oil–water separation, and for hazardous waste treatment. They have been used as high-temperature CO2 adsorbents and can provide thermal energy storage for solar power plants as well as heat recovery from cooling water from power plant turbines. Given the ubiquity and importance of the ceramics industry, its decarbonization is paramount. Decoupling ceramics from the negative societal and environmental impacts associated with their production requires an approach that considers the full set of sociotechnical systems involved in making, distributing and using this crucial material (see figure below).
Framing the ceramics industry as a sociotechnical system
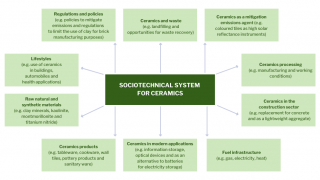
Options for decarbonizing the ceramics industry
Although energy efficiency in the ceramics industry has increased over the last few decades, energy costs still account for about 30 per cent of production costs and represent a substantial portion of production-related emissions. Improving energy efficiency through process optimization, materials reformulation for more efficient ceramics firing, improved energy management and fuel switching therefore offer economically viable routes for CO2 abatement. For example, a hybrid kiln that reuses exhaust gases to drive a heat pump can deliver up to 65 per cent in energy savings, while microwave-assisted drying and firing can deliver up to a 99 per cent reduction in energy use.
Nevertheless, other segments of the sociotechnical system also need to be addressed to effectively decarbonize the ceramics industry (see figure below). Optimizing inputs using circular economy principles can substantially reduce the need for raw material extraction as well as associated emissions. For example, the use of waste glass and paper sludge, both waste products of other industries, can deliver economic savings and significantly lower ceramic firing temperatures. Research also suggests that reusing and recycling ceramic waste – which accounts for about 50 per cent of demolition and construction waste – can lead to improvements in the quality of ceramic products while mitigating GHG emissions and reducing landfill waste. For example, using ceramic waste in mortars can deliver higher mechanical properties and lower water permeability. A low proportion of ceramic particles can also increase the mechanical strength of cement-based materials, such as mortars, while reducing process emissions associated with the cement industry.
Sociotechnical options for decarbonizing the ceramics system
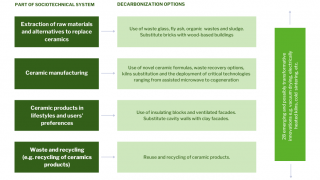
Despite the evident benefits of decarbonizing the ceramics industry, barriers remain. There are technical challenges to replacing fossil fuels without implementing entirely new technologies, some of which are unproven or untested at scale. Variation in the physical properties of clays from around the world means that localizing extraction is not always viable. Likewise, variations in local climatic conditions play an important role in determining the options for the decarbonization of ceramics production, and limit the applicability of technology transfer.
There are other barriers in terms of financial and economic disincentives, with some research suggesting that the dominant barrier to adopting low-carbon ceramic production technologies is related to the costs associated with the deployment of new technologies. The absence of information, specifically related to the lack of cost-benefit and viability analyses of efficient technologies, further impedes investment in more sustainable measures. Geographical location and the fact that many manufacturing sites are widely dispersed also influence the deployment of technologies. For instance, adopting low-carbon hydrogen for fuel switching is most likely to occur in industrial clusters where hydrogen production, distribution, and use are economically feasible. A lack of supporting standards can also work against the decarbonization agenda, recognizing, of course, that increased regulation can introduce additional costs to firms and hinder their competitiveness.
The ceramics industry does not operate in isolation and several social factors consequently need to be considered alongside the decarbonization agenda. Areas for future research from a sociotechnical perspective are therefore relevant. On the supply side, for instance, future research should focus on analyzing which regions have suffered the biggest ecological impacts from resource extraction for ceramics production. This type of analysis could be replicated for all materials used for ceramics manufacturing. On the demand side, there needs to be a greater understanding of consumer preferences, especially regarding construction materials; this could help to reveal whether more sustainable options can replace ceramics without compromising the desirability of the intended application. A sociotechnical approach offers a framework for understanding the various facets and interactions of the industry, the barriers to its decarbonization as well as the economic and environmental opportunities associated with decarbonization.
Disclaimer: The views expressed in this article are those of the authors based on their experience and on prior research and do not necessarily reflect the views of UNIDO (read more).
Have your say
What is your opinion on the IAP?